Childhood Cancer
Radiation therapy in children requires a delicate balance between efficacy and radiation-related late toxic effects. Acute radiation effects are similar to those occurring in adults; however, the heightened toxicity of combined chemoradiation therapy must be borne in mind because multiagent chemotherapy is often used in sequence or concurrently with radiation.
It is incumbent on the radiation oncology community to recognize the functional consequences of childhood cancer and its therapies. Of 142 children followed for 6 to 21 years after successful cancer management during the 1950s and 1960s, Li and Stone described major physical defects in 52%, but virtually all survivors were living “fully active lives.” Later series similarly indicate physical abnormalities in most long-term survivors, 40% of whom show functional deficits after childhood cancer therapy. Data from long-term survivors of Wilms’ tumor who were treated before they were 1 year old confirm the frequency of significant, largely musculoskeletal changes but the relative lack of life-threatening or disabling problems.
Growth disturbances are unique effects of cancer therapy in growing children, and they have been observed in approximately 40% of long-term survivors. Scoliosis, for example, had been identified as a consequence of inhomogeneous vertebral irradiation. Vertebral growth changes after current radiation techniques are identifiable although clinically insignificant at dose levels below 35 Gy.Reduction in vertebral height after radiation therapy at dose levels of at least 24 Gy is most apparent in children treated before they are 6 years old or during the adolescent growth spurt. Changes in soft tissue development often exceed changes in developing bone after modern radiation therapy. Muscular hypoplasia, for example, is common, resulting in functional or aesthetic alterations depending on the region irradiated.
Radiation changes in long bones include reduction in bone length resulting from treatment of epiphyseal growth centers.The apparent threshold for significant changes in bone length is age-related; changes can be anticipated after doses as low as 10 to 15 Gy in very young children (<3 years) and more than 25 Gy in children older than 3 to 5 years. Growth deficits are proportional to the amount of potential growth related to the irradiated epiphysis at the time of treatment. In the head and neck region, doses as low as 18 Gy are associated with observable defects in skull or facial bone development.10Orbital growth is altered after surgery plus radiation for retinoblastoma.
Alterations in growth also occur as a result of radiation-related endocrine dysfunction. Growth hormone deficiency has been identified in more than 50% of children who have undergone irradiation of the pituitary-hypothalamic region. A dose effect is apparent, with growth hormone deficits after doses above 25 to 30 Gy in children treated for primary brain tumors and sarcomas of the head and neck region. After 24 Gy of preventive cranial irradiation for acute lymphoblastic leukemia (ALL), subtle growth hormone deficits become apparent as the child undergoes the pubertal growth spurt. In girls, even low radiation doses (18 to 24 Gy for ALL) have been associated with early onset of puberty. Hypothyroidism also occurs relatively often after cranial irradiation; when the thyroid region is included within the radiation volume, clinical or chemical evidence of decreased thyroid function is apparent in 10% to 25% of children after doses of 18 to 26 Gy (e.g., for ALL or Hodgkin disease) and in more than 50% of children after doses exceeding 25 to 30 Gy (e.g., for medulloblastoma, sarcoma, or Hodgkin disease). Complicating matters are recent findings indicating that up to 50% of children with brain tumors have preexisting hormonal deficits before undergoing irradiation.
Other radiation-related somatic changes include late pulmonary deficits. These are caused primarily by diminished thoracic and lung parenchymal volumes after low-dose irradiation (15 Gy) in young children with Wilms’ tumor and after carmustine as a component of multimodality therapy in children with brain tumors.
The identification of CNS changes after cranial irradiation in conjunction with intrathecal or intravenous chemotherapy in ALL has led to relatively limited use of preventive cranial irradiation in this setting. A subacute syndrome of somnolence, often associated with nausea, irritability, or low-grade fever, has been documented in approximately 50% of children 4 to 8 weeks after cranial irradiation to 18 to 24 Gy. Cerebral atrophy and mineralizing microangiopathy are identifiable on computed tomography (CT) or magnetic resonance imaging (MRI) studies after treatment with cranial irradiation or chemotherapy. Leukoencephalopathy is a more profound, sometimes fatal entity histologically identified as focal coagulative necrosis of the white matter. The clinical syndrome includes lethargy, seizures, perceptual changes, and cerebellar dysfunction. The imaging diagnosis is based on characteristic diffuse white matter changes (Fig. 37-2). Although leukoencephalopathy is independently related to cranial irradiation and to methotrexate (predominately systemic but also intrathecal), its incidence is highest among patients treated with high-dose or prolonged intravenous methotrexate after cranial irradiation.
FIGURE 37-2
Transverse section (A) and coronal section (B) T2-weighted MRI scans reveal extensive white matter changes (arrow) characteristic of leukoencephalopathy in an 11-year-old child given high-dose methotrexate without irradiation for acute lymphoblastic leukemia. The imaging findings were associated with the onset of generalized seizures and lassitude, all of which improved over the ensuing 6 to 8 months.
Neuropsychological alterations have been well documented in survivors of ALL. Eiser initially described significant deterioration of the intelligence quotient (IQ) in children treated with cranial irradiation. Several subsequent studies have confirmed IQ declines related to cranial irradiation and to methotrexate administration. Treatment of children younger than 3 to 4 years generally has been associated with a greater risk of neuropsychological deficits.
Reproductive and genetic effects of abdominal irradiation include an increased risk of perinatal mortality and low-birth-weight infants. No excess congenital malformations or early malignancies in first-generation offspring have been described.
Perhaps the single most important issue affecting the risk-benefit ratio for radiation therapy in children is the frequency of secondary tumors. After orthovoltage irradiation, Li and colleagues reported a 10-year actuarial incidence of treatment-related second neoplasms approaching 14%. Although related to alkylating agents and radiation therapy, 90% of the tumors in Li’s initial study occurred within the radiation fields. The frequency of secondary tumors after megavoltage irradiation approximates 4% to 7% at 20 years. Secondary cancer is more common in girls than in boys, reflecting an incidence of radiation-related breast cancer of as much as 20% after the second decade after irradiation for pediatric Hodgkin disease.
Some findings suggest that certain disease entities, including Ewing’s sarcoma, are associated with a higher incidence of secondary cancer, especially sarcomas. An increased incidence of spontaneous and treatment-related neoplasms is apparent in children with genetically determined disorders such as neurofibromatosis, xeroderma pigmentosum, and Gorlin’s or basal cell nevus syndrome. The highest risk of secondary cancer is in children with the genetic form of retinoblastoma. In this group of young children with known familial disease or spontaneous bilateral disease, the chance of secondary tumors, largely but not entirely radiation-related bone sarcomas, has been 25% to 40% by 20 years after treatment and up to 50% by 50 years after irradiation (compared with only 5% in patients with similarly treated but spontaneous unilateral retinoblastoma). Fully one third of second malignant neoplasms after radiation therapy identified in the Late Effects Study Group occurred in patients with genetic disorders.
In considering the added benefit of irradiation in disease control, oncologists must always consider the potential for functional limitations and even death that follow therapeutic interventions in this often vulnerable age group. As the role for technically sophisticated radiation therapy is reexamined and often expanded, it is important to neither discount the importance of locoregional control, often best achieved with irradiation, nor ignore the late consequences of irradiation in young and developing children.
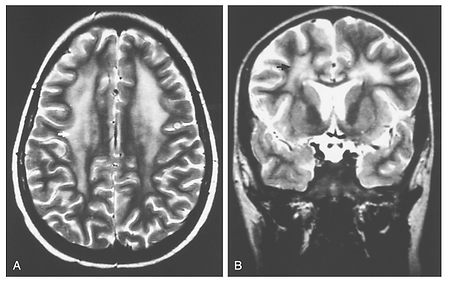